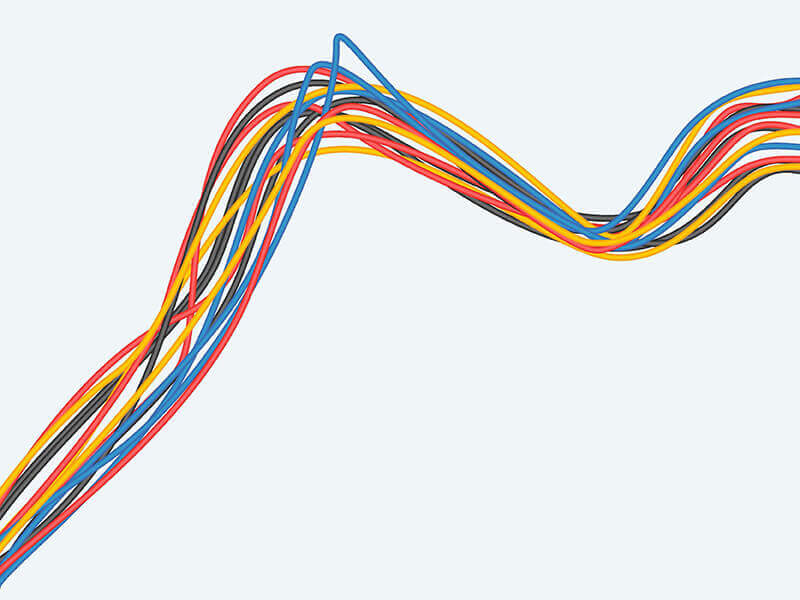
Pressure Sensor Cable
Overview of Pressure Sensor Cables
A pressure sensor cable is a critical element in any pressure-sensing system. Unassumingly, it carries the pressure data from the sensor to the monitoring equipment, adapting to its environment while overcoming physical constraints. Some primary features of these cables include:
1. Conductors:
Typically made of copper or tinned copper wires, these conductors excel in electrical conductivity and corrosion resistance, ensuring an efficient transmission of signals.
2. Insulation:
Encasing the conductors, insulators provide electrical separation and protection and are often constructed from PVC, TPE, or Teflon to deliver excellent electrical, thermal, and chemical resistance.
3. Shielding:
Incorporated to decrease electromagnetic interference (EMI) and radio frequency interference (RFI), shielding can be fashioned from copper braid or aluminum foil.
4. Jacket:
The outer covering of the cable offers mechanical and environmental protection. PVC, TPE, and Teflon are popular choices due to their flexibility, temperature resistance, and chemical resistance.
Importance of Pressure Sensor Cables in Various Applications
Pressure sensor cables are instrumental in conveying precise pressure data from sensors to control systems or monitoring devices, and their appropriate selection is crucial to ensuring accuracy and efficiency. Some compelling examples of their applications include:
In these systems, pressure sensor cables are vital in measuring and regulating parameters like refrigerant pressure, air pressure, and filter conditions. Selecting the right cable enables accurate and reliable data transmission, which translates to energy efficiency and peak system performance.
Relying on these cables, hydraulic systems can closely monitor pressure data from pumps, valves, and actuators. Given the high-pressure environments and demanding conditions, robust cables with superior electrical properties are critical to maintaining system efficacy, reliability, and safety.
In this context, pressure sensor cables are key to safeguarding the storage and transportation of gases like natural gas or hydrogen. Accurate pressure measurements are paramount in preventing over-pressurization, leaks or catastrophic failures. Consequently, these cables require high burst pressure ratings and resistance to harsh chemicals and extreme conditions.
Types of Pressure Sensor Cables
Pressure sensor cables form the backbone of countless sensing systems, ensuring precise communication between pressure sensors and monitoring devices. The right cable type is crucial for achieving peak performance, and so, we must explore the distinct characteristics of each option:
1. General Purpose Pressure Sensor Cables:
These cables cater to a wide array of applications, providing the essential features for reliable pressure data transmission. With a typical conductor construction of copper or tinned copper wires, general-purpose cables offer excellent electrical conductivity and corrosion resistance. They are well-suited for industrial automation, HVAC systems, and fluid power systems.
2. High-Temperature Pressure Sensor Cables:
When pressure sensing systems are exposed to extreme temperatures, the high-temperature pressure sensor cables come to the rescue.
Superior materials such as PTFE (Teflon) insulation, silicone jacketing, or glass fiber braiding provide heat resistance up to 260°C (500°F). These cables are indispensable for applications involving exhaust gas pressure monitoring in automotive or aerospace sectors, or processes in the petrochemical industry.
3. Submersible Pressure Sensor Cables:
Waterproof and resistant to chemicals, corrosion, and abrasion, submersible pressure sensor cables go above and beyond to deliver reliable performance in underwater environments.
Designed to withstand submersion depths up to 500 meters (1640 feet), these cables are ideal for underwater monitoring systems, submersible pumps, and even oceanographic research.
They may feature polyurethane or PVC jackets for added protection against the harsh marine conditions.
Choosing the right materials for cables is essential to ensure peak performance in specific applications. In doing so, we’ll examine the unique characteristics of each material, while providing helpful knowledge and relevant content.
Material Choices for Pressure Sensor Cables
Three key materials set the standard for pressure sensor cables: Polyvinyl Chloride (PVC), Polyurethane (PUR), and Polytetrafluoroethylene (PTFE). Each material presents its own advantages and challenges. In order to select the most suitable cable for your application, it’s crucial to understand how they differ.
1. Polyvinyl Chloride (PVC) Cables:
PVC is popular due to its low cost, high flame resistance, and reasonable electrical properties.
However, there are downsides, such as degradation under sunlight, lower chemical resistance, and reduced performance at high temperatures (typically up to 85°C/185°F). PVC cables are ideal for general-purpose applications like HVAC systems, indoor installations, and automation equipment.
2. Polyurethane (PUR) Cables:
PUR rises above PVC in terms of durability, abrasion resistance, and flexibility.
With added resistance to oil, weather, and exceptional low-temperature performance (down to -40°C / -40°F), PUR cables shine under harsh conditions. This makes them the go-to choice for heavy-duty industrial applications, construction sites, and outdoor installations.
3. Polytetrafluoroethylene (PTFE) Cables:
PTFE, or Teflon, is hailed for its exceptional resistance to chemicals, high temperatures, and extreme environments.
Capable of withstanding temperatures up to 260°C (500°F), PTFE cables are often chosen for use in the aerospace, petrochemical, and automotive industries. The only downside to PTFE is its higher cost, which may not be justified in standard applications.
4. Which is good for Seawater?
PVC is a more cost-effective option, its susceptibility to degradation in a seawater environment makes it less ideal for this application. PTFE, though more expensive, is likely to provide a more durable and reliable solution due to its superior chemical resistance and mechanical properties.
PUR could be a suitable choice for a seawater application, offering a balance between cost, chemical resistance, and mechanical durability. However, for long-term exposure to harsh seawater conditions, PTFE might still be the more reliable choice due to its superior chemical resistance. As always, it’s critical to consider the specific requirements and constraints of your application when making your choice.
In a seawater environment, PTFE’s excellent chemical resistance makes it an ideal choice. It can withstand the harsh conditions without degrading, ensuring the longevity and reliability of your level transmitter.
Therefore, if longevity and reliability in harsh conditions are a priority, then PTFE would be the better choice.
Below is a comparison table summarizing key material properties:
Industry | Cable Requirements | Material | Temperature Range | Chemical Resistance | Mechanical Strength | Environmental Factors | For water | For oil |
---|---|---|---|---|---|---|---|---|
Medical | Biocompatible, sterilizable, low noise, good signal transmission | PTFE(Teflon), Silicone | Varies depending on application | Good | Good | Cleanliness, flexibility | Excellent | Excellent |
HVAC | Wide temperature range, moisture resistance, chemical exposure | PVC, TPE | -40°C to +105°C | Moderate | Moderate | Moisture, UV resistance | Fair | Fair |
Oil/Gas | Extreme temperatures, high pressures, harsh chemicals | PTFE(Teflon), ETFE | -200°C to +200°C | Excellent | High | High pressure, temperature | Excellent | Excellent |
Deep Well Level Measurement | High pressures, moisture resistance, chemical exposure | PE, PUR | -40°C to +80°C | Good | Good | High pressure, moisture | Excellent | Excellent |
Hope above analysis should equip you with the vital information needed to choose the material for your pressure sensor cable application.
Selecting the Right Pressure Sensor Cable
We must consider several aspects to choose the most appropriate pressure sensor cable, including application requirements, environmental factors, cable length, flexibility, and consulting with manufacturers. Let’s examine these factors step by step, providing relevant content and helpful knowledge to support and guide your decision-making process.
1. Application requirements:
Assess the cable’s purpose, voltage, and current capacity needed. Consider load cell or strain gauge types, measurement range, accuracy, and temperature compensation requirements. This analysis will ensure that selected cables meet the application’s demands.
2. Environmental factors:
Evaluate the cable’s exposure to environmental conditions such as water, oil, chemicals, temperature fluctuations, and UV radiation. As discussed in our previous blog post on material choices for pressure sensor cables, these factors influence the cable material selection.
3. Cable length and flexibility requirements:
Determine the necessary cable length and evaluate if flexibility is critical, especially in confined spaces. Extended lengths may influence signal quality, so select cables with appropriate shielding, insulation, and conductor materials.
4. Consulting with manufacturers for custom solutions:
Collaborating with cable manufacturers could yield tailored solutions, meeting specific requirements or unique conditions. These solutions may involve custom cable designs, additional shielding, or specialized terminations.
Here is a summary of decision-making factors is found in the table below:
Factors | Considerations | Examples |
---|---|---|
Application Requirements | Voltage, | Load cells, |
Current capacity, | Strain gauges, | |
Sensor type, | Temperature compensation | |
Measurement range, | ||
Accuracy | ||
Environmental Factors | Exposure to water or oil, | PVC, PUR, PTFE cables |
Exposure to chemicals or others media, | ||
Temperature fluctuations, | ||
UV radiation | ||
Cable Length & Flexibility | Cable length, | Shielding, |
Signal quality, | Insulation, | |
Space constraints | Conductor materials | |
Consultation with Manufacturers | Custom cable designs, | Tailored solutions for unique requirements |
Additional shielding, | ||
Specialized terminations |
At last, taking into account these crucial factors will enable you to select the right pressure sensor cable confidently, ensuring optimal performance and reliability in your required application.
Cable Connectors and Termination Options
Let’s start with an understanding of the three most common connector types used in pressure sensor cables:
1. Circular connectors:
These types of connectors are widely used in various industries, particularly in harsh environments, as they offer excellent protection against environmental factors such as water and dust. Circular connectors often come in a variety of pin configurations and are generally built with durable materials such as brass or stainless steel, while the insulating body is usually made of plastic or rubber.
Notable circular connector series include the M12, M8, and 7/8″ connectors.
2. Terminal block connectors:
Terminal block connectors are an ideal choice for applications where ease of installation is a priority.
They are typically panel-mounted and consist of a series of terminal points, allowing for multiple sensors to be connected to a single terminal block. These connectors offer secure connections as wires are clamped down using screws or spring-loaded mechanisms, ensuring a dependable bond between the cable and the connector.
3. Direct wire:
In some cases, pressure sensors may not require any specific connector type and can be directly wired to the sensing device. This option is cost-effective and provides maximum design flexibility. However, direct wiring poses challenges when it comes to the maintenance and replacement of the pressure sensor.
Now, let’s explore the key mechanical and environmental considerations when selecting connectors for pressure sensor cables:
1. Operating temperature:
Depending on the application, pressure sensor cables may be exposed to extreme high and low temperatures. Therefore, ensuring that the chosen connector material can withstand these temperatures without experiencing degradation or failure is essential.
2. Ingress protection (IP) rating:
Connectors in harsh environments should have a high IP rating to ensure optimal protection against dust and water intrusion. Standard IP ratings include IP67, which offers protection against dust and temporary immersion, and IP68, which protects against dust and continuous immersion.
3. Chemical resistance:
In certain industrial environments, connectors may come into contact with corrosive chemicals. Selecting materials that can resist the chemicals’ damaging effects is crucial in these cases.
4. Vibration and shock resistance:
Since pressure sensor cables are often employed in dynamic applications, connectors should be designed to withstand vibrations and mechanical shocks without compromising their performance or reliability.
As promised, here’s a summary table that includes relevant data, numbers, and models for the cable connectors and termination options we’ve discussed so far:
Factor | Importance | Examples / Data |
---|---|---|
Operating Environment | Must withstand environmental conditions such as water, chemicals, dust, or humidity | IP ratings (IP67, IP68) |
Temperature Range | Must be compatible with the application's temperature range | -40°C to +125°C |
Chemical Compatibility | Must be resistant to chemicals it may come in contact with | Resistance to acids, bases, solvents |
Mechanical Stress | Must withstand tension, compression, bending, or abrasion | Tensile strength, flexibility |
Cable Length | Should be appropriate for the application, considering signal attenuation and installation | 1m, 5m, 10m, etc. |
Shielding Requirements | Reduce EMI if necessary | Shielded or unshielded cables |
Connector Types | Must be compatible with the pressure sensor and output device | M12, M8, DIN, et |
Cable Shielding and Grounding
Significance of shielding:
First, let’s begin by examining the importance of shielding in preventing electromagnetic interference (EMI): Pressure sensors can be sensitive to electromagnetic noise generated by nearby electrical devices, power lines, or even radio frequency transmissions. EMI can cause inaccurate sensor readings, leading to operational difficulties and unreliable performance. Shielding effectively minimizes EMI and ensures precise and reliable data from pressure sensors.
Techniques for improving noise immunity
Now, let’s discuss some techniques for improving noise immunity:
1. Twisted pair cables:
Using twisted pair cables can significantly reduce the impact of EMI. The twisting of the conductors equalizes the exposure to external electromagnetic fields, effectively canceling out noise and increasing noise immunity.
2. Shielding materials:
Implementing proper shielding materials is essential in minimizing EMI. Common shielding materials include copper, aluminum, and steel. The choice of shielding material depends on several factors, including the frequency range of the electromagnetic noise and overall cost constraints.
3. Shielding effectiveness:
Achieving optimal shielding effectiveness requires a balance between the conductivity of the shielding material, its thickness, and the frequency of the electromagnetic noise. Higher conductivity materials generally provide better shielding effectiveness.
Grounding best practices for pressure sensor cables
Finally, let’s explore grounding best practices for pressure sensor cables:
1. Single-point grounding:
Providing a single-point ground for the shielding material is essential to minimize ground loops and reduce noise. This ensures any EMI absorbed by the shield is properly directed to ground.
2. Proper grounding techniques:
When grounding the shielding, it is crucial to ensure a low impedance connection to ground. This can be achieved by using short and wide grounding straps or large surface area grounding pads to provide minimal resistance.
3. Regular inspection and maintenance:
Regularly inspect and maintain the grounding connections for pressure sensor cables to ensure proper shielding effectiveness and prevent potential issues due to corrosion, loose connections, or damage to the shielding.
Continue: Pressure Sensor Cable part 2/2